
Fundamental Principles of Organic Reactivity
We are interested in conceptualizing new principles of organic reactivity and catalysis, both experimentally and computationally.
Website of the Guanqi Qiu Group

The critical factors of chemical reactivity have been the interplay of rate and driving force. The free energy of activation can be expressed by a combination of the intrinsic barrier (activation Gibbs energy at zero driving force) and the reaction Gibbs energy. While there are numerous studies on the rate-driving force relationship and intrinsic barrier, the vast majority are concerned with and stop at finding out the quantitative rate-driving force relationship and the intrinsic reactivity. Intentional attempts to systematically modulate intrinsic reactivity are very rare. Our research focuses on delving deep into the core principles that govern intrinsic properties of reactions, aiming to revolutionize how we understand and manipulate them. Our research programs centered on enabling the systematic modulation of intrinsic reactivity, and applying it in formulating new principles of reactivity and selectivity manipulation in catalytic reaction design.
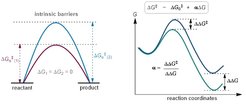
Modulate Intrinsic Reactivity

The intrinsic barrier is considered to reflect the reorganization energy required for the deformation of the nuclear coordinates of the reactant state to that of the product state. The Brønsted slope α indicates the sensitivity of the reaction rates to changes in driving force. Product stabilization or destabilization factors (e.g., conjugation, solvation, steric hindrance, aromaticity, etc.) could advance or lag behind primary changes in bonding at the transition state, resulting in different Brønsted slope α values. We aim to formulate a methodology that systematically modulates intrinsic reactivity and sensitivity of rate driving force dependence, making them in control.
Utilize Intrinsic Reactivity
By manipulating intrinsic reactivity, we are working on designing advanced catalytic reactions to steer organic synthesis in innovative directions. We will utilize the formulated methodology of systematic modulation of intrinsic reactivity to design catalytic reactions.
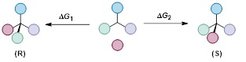
We aim to translate the intrinsic barrier and the sensitivity of the rate-driving force dependence into the concrete features of the catalytic systems for organic synthesis. By doing so, we can intuitively identify specific chemical features of these catalytic systems, allowing us to manipulate reactivity and selectivity effectively through straightforward adjustments to the driving force.
Explore Quantum Mechanical Tunneling under Ambient Conditions
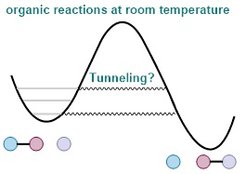
Quantum Mechanical Tunneling (QMT), a through-barrier process despite an energy insufficiency to surmount them, has recently gained recognition in chemistry. QMT not only significantly enhances reactivities, but can also be a selectivity control element of chemical reactions, thus unveiling new possibilities for reactivity. To fully explore the vast potential of QMT in synthesis, we are interested in actively searching for QMT processes under ambient conditions. One prevalent method for detecting QMT involves checking for temperature independence of reactivity. However, this is true only at very low temperatures, where excited vibrational states are not populated, and tunneling can only occur from the ground state. Another approach relies on very large kinetic isotope effects (KIEs). By substituting a heavier isotope for one of the atoms involved in the reaction, the tunneling reaction rate decreases drastically. While effective for hydrogen atom tunneling diagnosis, the KIE is much smaller for heavy-atom tunneling and would possibly be attenuated by other perturbations and experimental errors, making the diagnosis less effective.
We will introduce a new diagnosis method for QMT processes that overcomes the limitations previously mentioned, making it feasible to detect QMT events under standard bench-chemistry conditions. By developing this new approach, we seek to broaden the scope of reactions that can be susceptible to QMT processes, ultimately paving the way for catalyzing QMT reactions. We aim to generalize the types of reactions that are prone to QMT phenomena, thereby providing valuable insights into the factors that govern these processes.